Adipose-Derived SVF Therapy: Step-by-Step Guide for Isolation, Storage, and GMP Compliance
- Admin
- 11 minutes ago
- 8 min read
The Stromal Vascular Fraction (SVF), derived from human adipose tissue, represents one of the most promising biologics in regenerative medicine. Rich in multipotent cell populations, including mesenchymal stem/stromal cells (MSCs or ASCs), pericytes, endothelial progenitor cells, and immune cells, SVF supports tissue regeneration through paracrine signaling, neovascularization, and immunomodulation.
Its applications continue to grow, extending from orthopedics and wound healing to aesthetic medicine and reconstructive surgery. However, despite its clinical value, one of the main challenges facing widespread SVF use is the absence of a globally accepted standard for its collection, processing, preservation, and release. A 2025 review by Cremona and colleagues offers clinicians and researchers a highly practical, step-by-step look at what it takes to create a GMP-compliant, high-quality SVF product that meets both regulatory and therapeutic standards.

From Liposuction to Laboratory: Standardized Harvesting and Transport
The journey of SVF begins with the collection of adipose tissue, most commonly via tumescent liposuction using manual cannulas or power-assisted devices like PAL™. Although harvesting methods can vary slightly between clinicians, this review confirms that the biological quality of SVF is less dependent on the liposuction technique and more dependent on how the tissue is processed afterward.
Clinical data from nearly 300 procedures showed that although the volume of adipose tissue collected may differ from surgeon to surgeon, cell yield and viability remained remarkably stable when processed under standardized conditions.
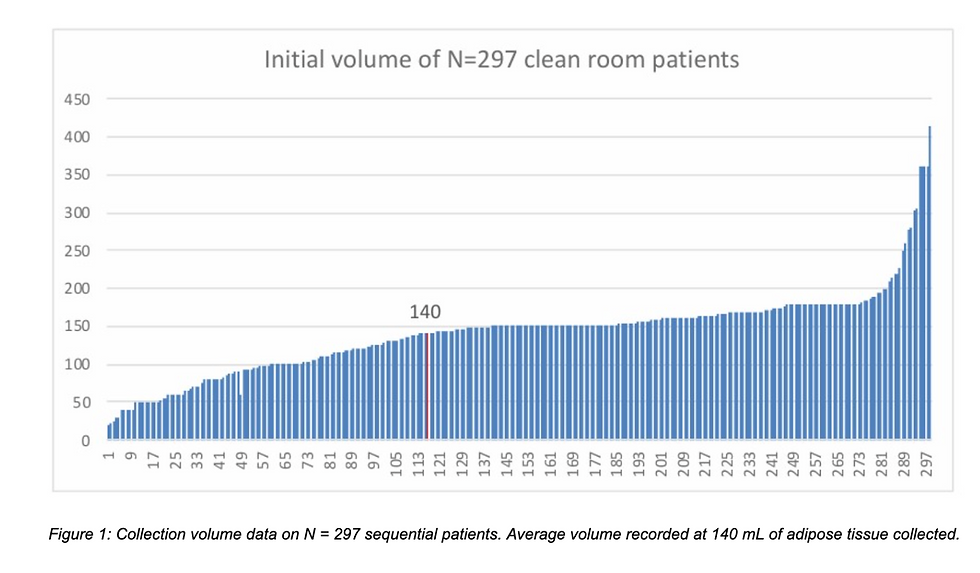
This figure demonstrates the average volume of lipoaspirate collected across 297 patients, emphasizing consistency across providers despite procedural variability.

The following set of figures showcases total nucleated cell counts and viability from over 300 SVF samples, with no significant deviation between different clinicians—reinforcing the importance of downstream consistency in processing rather than upstream harvesting technique.
Transport of lipoaspirate is another critical phase. For optimal viability, adipose tissue should be processed within 24 hours, stored in sterile containers, and transported at a controlled 4°C to the GMP lab. These steps preserve both the stem cell integrity and the downstream regenerative properties of the SVF product.
The method of liposuction, whether manual or power-assisted, does not significantly affect the quality of the harvested SVF, as long as the downstream processing is standardized. What proves to be more critical is maintaining consistency in the transport conditions, particularly with regard to time, temperature (ideally around 4°C), and sterility during transit from the surgical site to the laboratory. When these parameters are controlled, both cell viability and total nucleated cell (TNC) counts have been shown to remain stable, regardless of the surgeon performing the procedure.
The 3 Isolation Methods: Enzymatic, Mechanical, or Hybrid?
The quality and functionality of stromal vascular fraction (SVF) largely depend on how it is isolated from adipose tissue. Isolation methods directly influence cell yield, viability, safety, and regulatory classification. The research by Cremona et al. outlines three main approaches currently used in both research and clinical practice: enzymatic digestion, mechanical isolation, and hybrid systems. Below is a detailed overview of each method, including their steps, outcomes, and practical considerations.
Enzymatic Digestion
Enzymatic digestion is considered the gold standard for SVF isolation in terms of cell yield and population diversity. This method uses collagenase, a proteolytic enzyme, to digest the extracellular matrix of adipose tissue, allowing stromal cells to be released into suspension.
Yields: 2.3×10⁵ – 1.8×10⁶ cells/mL
Viability: 70–99%
Downside: Classified as an ATMP (Advanced Therapy Medicinal Product), requiring GMP compliance.
Steps Involved:
Harvesting: Lipoaspirate is collected via tumescent liposuction and placed in a sterile container.
Washing: The adipose tissue is washed with buffered saline to remove blood and anesthetic residues.
Enzymatic digestion: Collagenase (Type I, II, or V) is added to the tissue and incubated at 37°C for 30–60 minutes, depending on protocol.
Centrifugation: After digestion, the mixture is centrifuged to separate the stromal vascular pellet from oil, adipocytes, and enzyme solution.
Washing and resuspension: The SVF pellet is washed to remove residual collagenase and reconstituted in saline or media.
Filtration and quality control: The final cell suspension is filtered and tested for sterility, viability, and cell count.
Mechanical Isolation
Mechanical isolation is a non-enzymatic method that uses physical disruption (e.g., shear force, centrifugation, filtration, or vibration) to separate stromal cells from adipose tissue. This method avoids the use of enzymes and is classified as a "minimally manipulated" process in many regulatory settings, making it more accessible for clinical application.
Yields: 0.3×10⁴ – 2.67×10⁶ cells/mL
Viability: 46–97.5%
Advantage: Considered “minimally manipulated”, enabling easier clinical adoption.
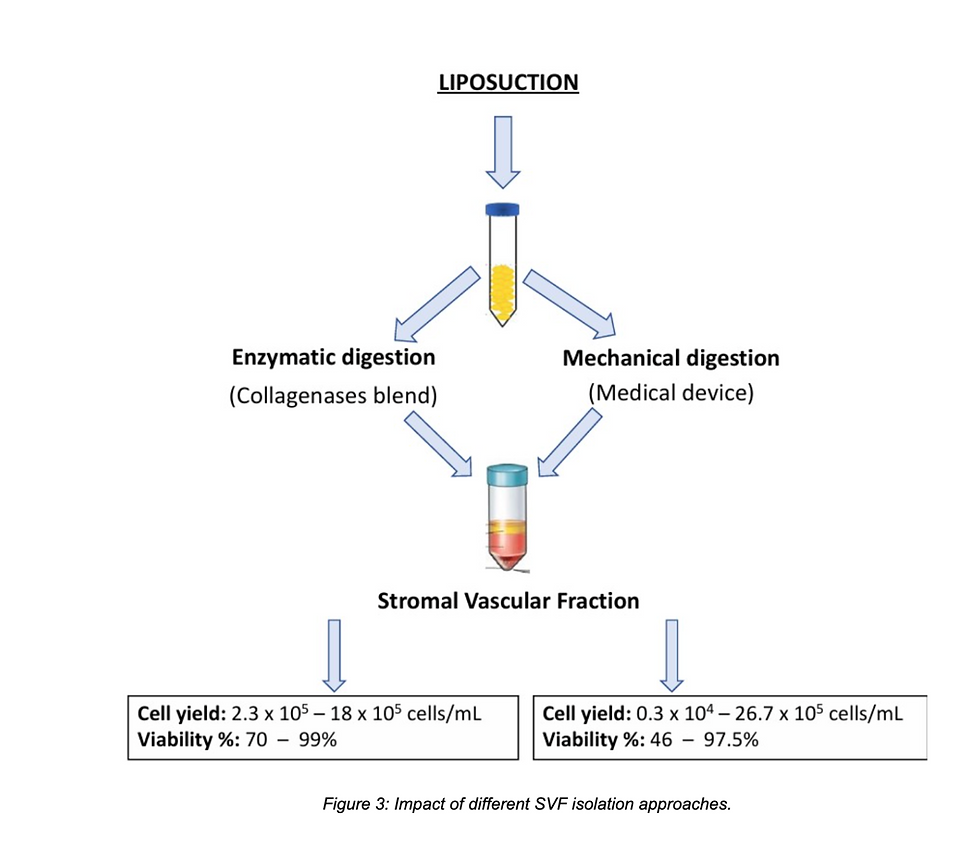
This figure compares over 20 documented protocols, showing that while enzymatic methods generally produce higher yields, mechanical methods can retain more extracellular matrix, which may aid in wound healing and tissue integration.
Steps Involved:
Harvesting: Adipose tissue is collected using standard liposuction techniques.
Emulsification or mechanical disruption: The fat is mechanically processed—this may include:
Passing the lipoaspirate repeatedly between syringes through connectors,
Using rotating blades or beads to break up tissue
Applying vibration, microfluidics, or ultrasound
Centrifugation or filtration: The disrupted tissue is centrifuged or filtered to isolate the stromal component from fat and fluid.
Collection of SVF: The lower layer or filtered portion contains the mechanically isolated SVF, rich in ASCs, pericytes, and ECM fragments.
Hybrid Systems
Hybrid approaches are also emerging, combining ultrasonic energy or mild enzymatic agents with mechanical disruption to maximize yield while preserving tissue microarchitecture. These approaches may become the future standard, offering both efficiency and safety.
Types and Strategies:
Ultrasound + Enzyme: Uses low-frequency ultrasound to pre-disrupt adipose tissue before applying a low concentration of collagenase, reducing digestion time.
Microblade + Enzyme: Physical disruption is performed first (e.g., with fine mesh or blades), followed by short enzymatic digestion.
Automated devices: Closed systems that integrate both mechanical and enzymatic steps, offering standardized protocols under semi-automated conditions.
Below is a summary of the 3 isolation methods.
Enzymatic digestion: Highest yield, high purity, but requires GMP compliance and regulatory oversight.
Mechanical isolation: Easier to deploy clinically, less regulated, retains ECM but with variable yields.
Hybrid systems: Combine the best of both—being optimized for yield, structure, and regulatory feasibility.
Cryopreservation: Banking SVF for Future Therapeutic Use
Cryopreservation is a powerful tool that allows SVF to be stored long-term while preserving cell viability. This enables clinicians to harvest adipose tissue once and use it for multiple treatments over time, reducing patient burden and maximizing therapeutic flexibility.
In the reviewed literature, several cryopreservation strategies are discussed, each with distinct methods for protecting cellular integrity during freezing and thawing. Below are the main approaches highlighted in the study by Cremona et al.:
Slow Freezing (Controlled-Rate Freezing)
This is currently the most widely adopted method for SVF preservation. It involves gradual cooling at approximately 1°C per minute, allowing water to leave cells slowly, thereby minimizing intracellular ice formation that can rupture membranes.
The standard cryoprotective medium typically includes:
Dimethyl sulfoxide (DMSO): A permeating cryoprotectant that stabilizes intracellular structures during freezing.
Human serum albumin (HSA): Added for osmotic balance and protein support.
This combination has shown consistent results in maintaining post-thaw viability above 70%, which is considered clinically acceptable for cell-based applications.
Advanced Cryoprotective Additives
To further enhance preservation, novel strategies are being explored. These include the use of nanomaterials and antioxidant agents to reduce oxidative stress during freeze-thaw cycles:
Nanographene oxide has been studied for its thermal conductivity and anti-oxidative properties, potentially minimizing cryoinjury.
Hydrogen gas and Vitamin E derivatives may also help protect cell membranes by reducing reactive oxygen species (ROS) during thawing.
These advanced additives are still under investigation but show potential in improving both viability and functional recovery of SVF cells.
Vitrification and Nano-Vitrification Techniques
Vitrification refers to ultra-rapid freezing that avoids ice crystal formation entirely by turning water inside cells into a glass-like solid. While this method is commonly used in oocyte and embryo preservation, applying it to SVF requires more nuanced protocols due to cell heterogeneity.
Nano-vitrification, a refinement of this approach, uses nanoparticles to distribute cold evenly and prevent ice nucleation at a cellular level. This strategy is being explored as a future alternative to standard slow freezing, potentially offering higher preservation fidelity for delicate cell populations such as pericytes or endothelial progenitors.
Cryopreserved stromal vascular fraction (SVF) has been shown to maintain both its cellular functionality and regenerative potential, making it a valuable option for long-term therapeutic use. The current standard for cryopreservation involves the use of dimethyl sulfoxide (DMSO) combined with human serum albumin (HSA), which helps protect cell membranes during freezing. Additionally, emerging techniques such as nano-vitrification are being explored to further improve post-thaw viability and extend the shelf life of SVF products.
Quality Control in GMP Settings: What Every Facility Needs to Monitor
Quality assurance is central to SVF processing—particularly under GMP conditions. Every batch must be tested for sterility, potency, viability, and safety before clinical release. Sterility testing is often done using Bact/Alert™ systems or LAL-based endotoxin detection (including recombinant kits like PyroSense™). Environmental monitoring must also follow strict Eudralex Annex 1 guidelines to ensure cleanroom compliance.
Cell viability is usually measured by flow cytometry, Trypan Blue exclusion, or automated fluorescence counters, and must meet defined thresholds (commonly >70%). Also critical is the Total Nucleated Cell (TNC) count, which correlates with therapeutic potency. For deeper functional insight, Colony Forming Unit (CFU-F) assays may be used to assess the presence of mesenchymal progenitors.
Good Manufacturing Practice (GMP) protocols require that every batch of stromal vascular fraction (SVF) undergo rigorous testing to ensure sterility, cell viability, and functional potency. Standardized metrics such as total nucleated cell (TNC) count and colony-forming unit–fibroblast (CFU-F) assays are commonly used to assess the regenerative capacity of the product. In addition to product-specific testing, consistent environmental monitoring within the laboratory is essential to maintain safety, minimize contamination risks, and uphold the overall reliability of SVF processing.
SVF Cell Profiling: Flow Cytometry for Clinical Precision
Profiling SVF is not just about quantity—it’s about identifying the right cells. The review outlines a validated flow cytometry panel developed by the Swiss Stem Cell Foundation and University Hospital of Marseille. This approach distinguishes between CD45+ hematopoietic cells (e.g., immune and blood cells) and CD45− stromal components (e.g., ASCs, pericytes, and endothelial cells).
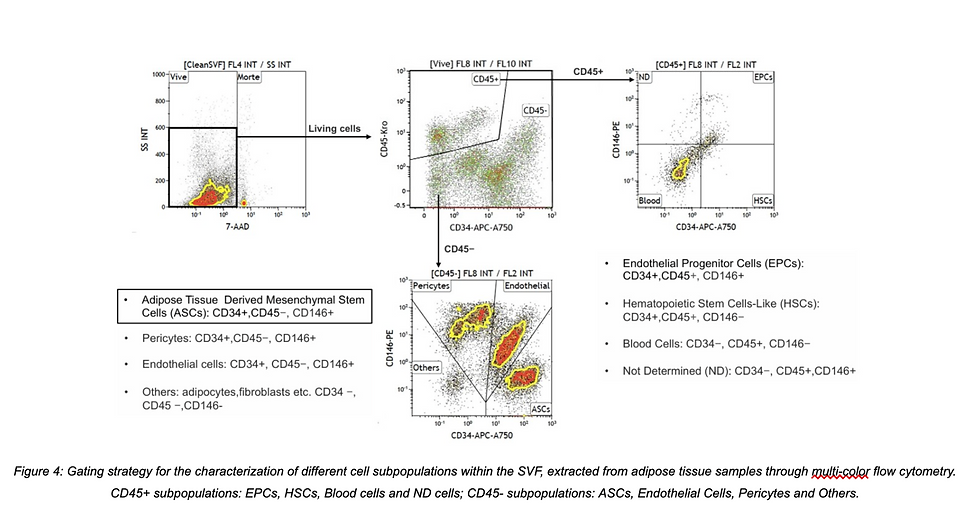
This figure illustrates a refined gating strategy, highlighting subpopulations such as:
CD34+CD146− ASCs
CD34+CD146+ endothelial progenitors
CD146+CD34− pericytes
These markers allow for greater standardization of SVF products, enabling clinicians to select or monitor specific cell populations based on their therapeutic needs.
Flow cytometry plays a critical role in ensuring the consistency and reliability of SVF products by enabling in-depth cell profiling. By analyzing specific surface markers—such as CD34, CD45, and CD146—clinicians and researchers can accurately identify and quantify functional subpopulations like mesenchymal stem cells, endothelial progenitors, and immune cells. The use of standardized gating strategies further enhances the reproducibility of results across different centers and laboratories, laying the foundation for future regulatory alignment and broader clinical adoption.
Conclusion
SVF represents one of the most versatile and potent biologics in modern regenerative therapy. This review lays out a clear, structured path from adipose harvesting to final injection—highlighting the protocols, cell biology, and regulatory considerations that make SVF both effective and safe for clinical use.
By adopting standardized methods for isolation, cryopreservation, quality control, and characterization, clinicians and cell-processing facilities can unlock the full potential of SVF—not just in aesthetic procedures, but across wound healing, orthopedics, and even organ repair.
Reference:
Cremona, M., Palma, E., Viganò, M., Veronesi, E., & Alessandri, G. (2025). Isolation and standardization of the stromal vascular fraction from adipose tissue for clinical applications in regenerative medicine: Current procedures and regulatory issues. Biomolecules, 15(2), 166. https://doi.org/10.3390/biom15020166
Learn techniques behind Anti-Aging Stem Cell Therapy
in our upcoming Mini Fellowship:
IFAAS Mini-Fellowship (Hands-On)
Anti-Aging Stem Cell Therapy
June 23, 2025 - Tokyo, Japan - [Register Now]
August 20, 2025 - Seoul, South Korea - [Register Now]
More Upcoming Global Events
Comments